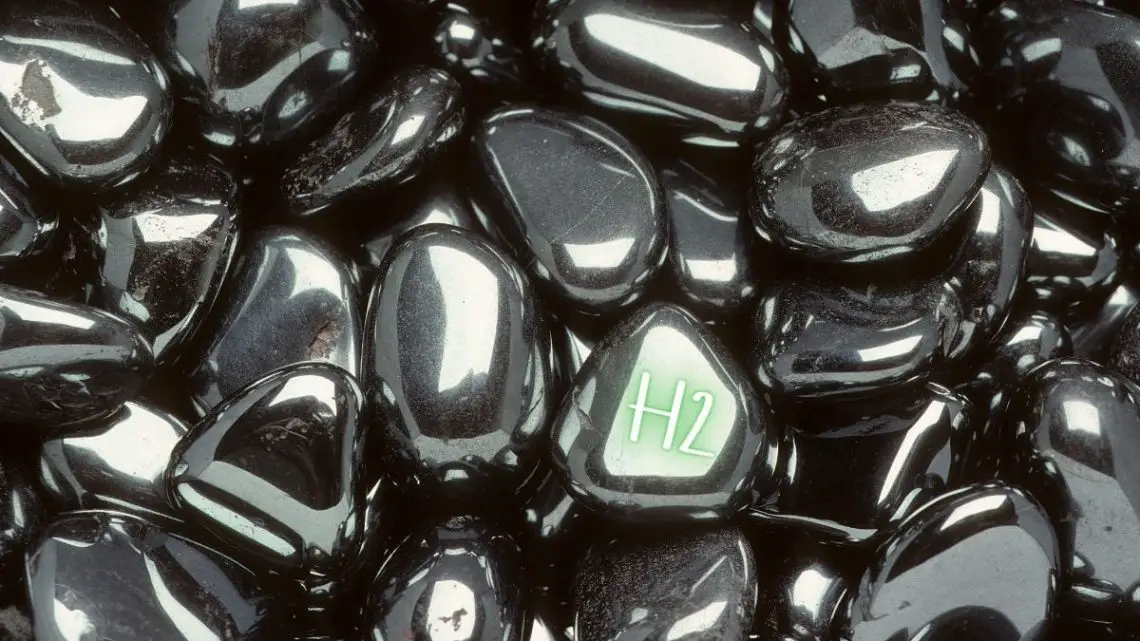
Hematite’s Hidden Power: A Green Hydrogen Breakthrough
July 21, 2024In an unexpected breakthrough, researchers at the Ulsan National Institute of Science and Technology (UNIST) have unlocked a new potential for hematite, a common form of iron oxide, transforming it into a highly efficient tool for producing green hydrogen. This discovery could significantly alter the landscape of green energy production.
An Unusual Twist in Green Hydrogen Research
Traditionally, hematite has been dismissed as a poor candidate for hydrogen production due to its subpar electrical performance. However, the UNIST research team, led by Professor Jang, has managed to alter this perception by making critical modifications at the microscopic level.
The Innovation: By incorporating trace amounts of germanium, titanium, and tin into hematite and subjecting it to heat treatment, the researchers created a nanoporous structure with pores smaller than 10 nanometers. This adjustment vastly improved hematite’s electrical conductivity and increased the surface area available for reactions.
The Result: This innovative approach led to a 3.2-fold increase in hydrogen production efficiency using sunlight. More impressively, the enhanced efficiency was sustained for over 100 hours without degradation, showcasing the stability of the process.
What is Hematite?
Yes, hematite is a form of rust. Hematite is an iron oxide mineral that forms when iron is exposed to oxygen and water, which is the same process that creates rust. Known scientifically as Fe₂O₃, hematite is an iron oxide compound that is abundant across the globe. This mineral can be found in various geological settings, including sedimentary, metamorphic, and igneous rocks. Its formation is often associated with the presence of water.
For instance, iron from hematite can precipitate out of water and accumulate in layers at the bottom of lakes, springs, and other standing bodies of water. Additionally, hematite is frequently present in banded iron formations, which are significant sources of iron ore. Its widespread occurrence and fundamental role in iron cycling make hematite an essential mineral in both natural and industrial contexts.
Deconstructing The Process: Science News and Harnessing Sunlight to Produce Hydrogen from Water
Photoelectrochemical (PEC) Water Oxidation
PEC water oxidation uses sunlight to generate hydrogen from water, specifically on hematite photoanodes. However, hematite traditionally faces significant challenges in this process.
Challenges with Hematite Photoanodes
Hematite has limited hole diffusion length and poor electrical properties, posing difficulties in efficient hydrogen production.
Innovative Solution by UNIST Researchers
UNIST researchers have overcome these obstacles by developing a highly porous structure via the Kirkendall effect at the interface of the overlayer and hematite precursor. They also fabricated branched hematite precursors that resulted in a nanoporous structure.
Nanoporous Structure Details
The new structure has pores smaller than 10 nanometers and an average strut diameter under 10 nm between pores, enhancing the material’s properties.
Morphological Engineering and Doping
To further improve the electrical properties of hematite, the researchers incorporated suitable dopants. The selection of these dopants was optimized using density functional theory.
Optimized Photoanode Performance
Using a NiFe(OH)x cocatalyst, the optimized photoanodes achieved a maximum photocurrent density of 5.1 mA cm⁻² at 1.23 VRHE, marking a 3.2-fold increase over the reference.
Significant Advancement
This significant improvement in PEC performance is attributable to the nanoporous structure combined with optimal doping conditions, representing a major advancement in hematite-based photoanode technology.
Expert Insights
Professor Jang underscored the profound implications of their research, marking it as a pivotal advancement toward the commercialization of green hydrogen production. He highlighted that this breakthrough could significantly influence its integration into a diverse array of semiconductor systems.
The research team noted, “Our findings demonstrate the potential of our strategy for developing highly nanoporous structures and suggest its applicability to a broad range of materials for various applications relying on surface reactions, including solar conversion, energy storage, and sensors.”
Impact on Green Energy Production
This breakthrough has far-reaching implications for the field of green energy production:
- Cost Reduction: By making hematite a viable option for high-efficiency green hydrogen production, the cost associated with producing green hydrogen can be significantly lowered. This makes it a more competitive alternative to fossil fuels.
- Commercial Scalability: The increased efficiency and stability demonstrated by the UNIST team pave the way for commercial-scale green hydrogen production. This is critical for achieving global carbon reduction targets.
- Sustainable Materials: Utilizing hematite reduces the dependence on rare and expensive metals often used in hydrogen production technologies, promoting sustainability.
- Advancements in Green Technology: The approach provides a model for enhancing surface reaction efficiency, potentially leading to advancements in other areas of green energy production, such as solar energy conversion and energy storage.
Looking Forward
The UNIST team’s research shines a light on the untapped potential of common materials in advancing green technologies. By transforming hematite into a highly efficient tool for hydrogen production, they have not only challenged existing perceptions but also opened new avenues for sustainable energy solutions.
For green technology innovators, this breakthrough represents a significant milestone. It underscores the importance of innovative approaches in overcoming existing limitations and achieving greater efficiencies in green energy production.